Advanced Learning Content is exclusively available via this website
Injury to the Airway and Lungs
Burns can also damage the airway and lungs, with life-threatening consequences. Inhalation injury of hot, smoked-filled air has three components, each of which can present alone or in any combination. They are: upper airway injury, lower airway injury (true smoke inhalation) and metabolic poisoning. Airway injuries occur when the face and neck are burned; the significance of being trapped in an enclosed space (burning room or car) cannot be underestimated.
Warning signs of burns to the respiratory system
- Burns around the face and neck, blistering inside the mouth
- A history of being trapped in an enclosed space
- Change to/hoarseness of voice
- Stridor
- Singeing of facial and nasal hair
Physical burn injury to the airway above the larynx
The upper airway extends from the lips to the vocal cords of the larynx. The structures of the larynx form an excellent heat sink and, similar to what happens during drowning, the vocal cords will close in an effort to prevent the ingress of hot gas into the lower airway. As a result, injuries above the larynx are thermal in nature. The mucosae of the oro-, naso- and laryngopharynx, and the upper surface of the vocal cords, are burned and the response is very similar to that seen under thermal cutaneous injuries, namely the movement of fluid from vessels into the extravascular space and oedema formation. The bony or cartilaginous skeletons of this part of the airway, however, do not allow the pressure of the swelling to be dissipated outwards; as such the airway begins to narrow as the mucosa swells into the lumen.
Lower airway injury
The lower airway extends from the underside of the vocal cords up to, and including, the alveoli. Lower airway injury is ‘true’ smoke inhalation injury. It is present in about 22% of all burn presentations but in 60% of those where central facial burns are present. At least 30% of patients with smoke inhalation injury die, compared with 2% of those without this type of injury, illustrating the deleterious impact of low airway injury. Smoke is a heterogeneous compound unique to each fire; as such, its components vary. Those capable of causing damage include heat, particulates, systemic toxins and respiratory irritants.
Heat
Although burns to the nasal and oropharyngeal mucosae are common, it is rare to encounter thermal injury below the vocal cords because heat exchange in the upper air passages is highly efficient. The exception is a hot steam injury, which contains a significant amount of latent heat; the respiratory epithelium rapidly swells and detaches from the bronchial tree, resulting in pulmonary casts.
Particulates and irritants
The chief contributor to the pathophysiology of smoke inhalation is particulate matter (Figure S46.1). Particles of soot carry a variety of toxins, sometimes as far as the alveolar level, suspended in air. The products generated during a fire comprise many different chemicals, including aldehydes, nitrogen oxides, halogen acids, sulphur dioxide, ammonia and phosgene. Those that are water soluble (such as ammonia and hydrogen chloride) dissolve in water in the airway mucous membranes, producing strong alkalis and acids, which elicit intense and prolonged inflammatory reactions at more proximal levels of the respiratory system. Poorly water-soluble compounds (such as phosgene, the aldehydes and the oxides of nitrogen) may cause severe delayed damage via late interaction with distal airway tissues (up to 48 hours after exposure). This is particularly important when treating patients who present early with apparently mild clinical effects after smoke inhalation.
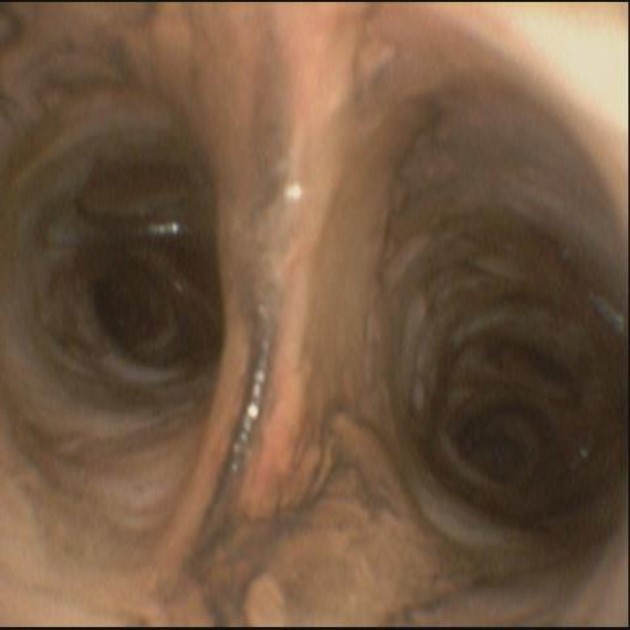
Figure S46.1
Bronchoscopy demonstrating soot in the trachea and bronchi.
Inflammation and Circulatory Changes
The circulatory changes initiated by a burn injury are complex and multifactorial, originating from both the actual injury of burned skin (eschar) and the inflammatory cascade. It is governed by a complex series of events. The release of neuropeptides and the activation of complement are initiated by the stimulation of pain fibres and the alteration of proteins by heat. The activation of Hageman factor initiates a number of protease-driven cascades, altering the arachidonic acid, thrombin and kallikrein pathways. Fluid is lost from capillaries and oedema formation occurs.
The shock reaction after burns
- Burns produce an inflammatory reaction
- This leads to vastly increased vascular permeability
- Water, solutes and proteins move from the intra- to the extravascular space
- The volume of fluid lost is directly proportional to the area of the burn
- Above 15% of surface area, the loss of fluid produces shock requiring resuscitation
Inflammatory response
The release of neuropeptides and the activation of complement are initiated by the stimulation of pain fibres and the alteration of body proteins by heat.
At a cellular level, complement causes the degranulation of mast cells and coats the proteins altered by the burn. This in turn attracts neutrophils, which also degranulate, with the release of large quantities of free radicals and proteases. Mast cells also release primary cytokines such as tumour necrosis factor alpha (TNFα), which act as chemotactic agents for inflammatory cells and propagate secondary cytokine release. These inflammatory factors alter the permeability of blood vessels and consequently large protein molecules move to the extravascular space. The damaged collagen and proteins, which unfold to form an increased surface area, further drive the oncotic pressure within the burned tissue, resulting in increased fluid shift from the intravascular to the extravascular space (Figure S46.2).
The role of burn eschar
The volumes of fluid required in the first 24 hours after injury to resuscitate patients with burns and to maintain core volume and peripheral perfusion are enormous; by some calculations, they are 100-fold greater than can be explained by alterations to the Starling forces alone.
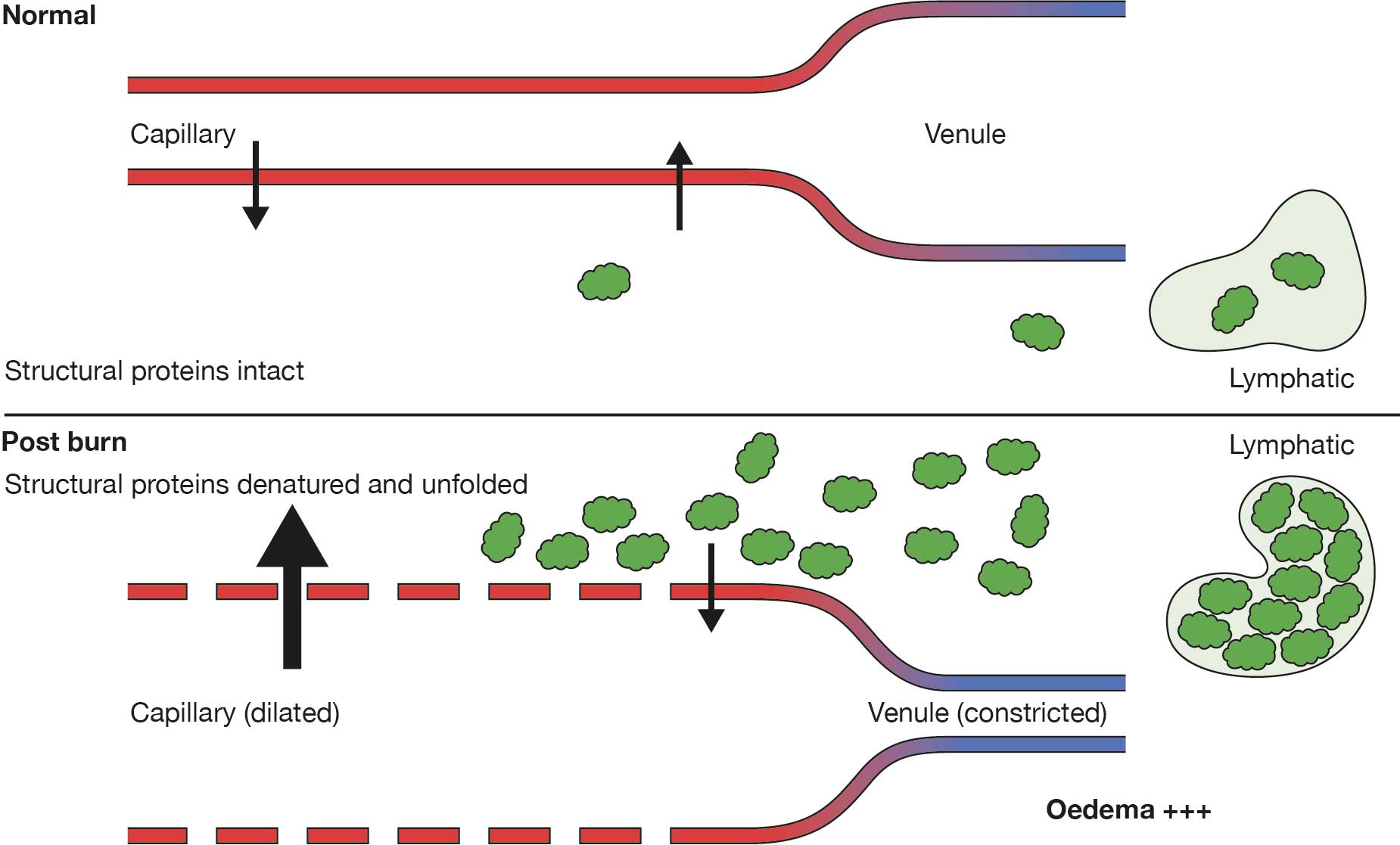
Figure S46.2
Capillary changes caused by burn injury. The green blobs shown are proteins.
Part of the explanation for this lies in interstitial compliance, which does not appear as a factor in Starling’s original equation. The molecular structure of the dermis (predominantly collagen types I and III and several glycosaminoglycans including hyaluronic acid) exerts hydraulic forces that stabilise the interstitial space while in their normal ‘coiled’ configurations. The destruction of the dermal macromolecules after deep burn injury alters the osmotic force in the dermal interstitium (because fragmentation increases the number of particles and ‘uncoiling’ allows expansion and reduces density), creating a highly negative interstitial tissue hydrostatic pressure (of the order of 19 mmHg for each milliosmole of solution). This could have the effect of creating an interstitial hydrostatic pressure of up to –40 mmHg according to some authors, but as low as –135 mmHg by other experimental models. Since positive interstitial hydrostatic pressure is one of the effectors of fluid movement into the vasculature, a markedly negative value creates a ‘sucking’ force moving potentially large volumes of fluid from the vasculature and into the interstitial space (Figure S46.3).
Starling forces: changes
Jv = Kf
([Pc – Pif] – σ[πc – πif])
- Kf 2- to 3-fold ↑, immediately, vasodilatation and ↑ capillary permeability
- Pc in burn: 2× ↑ over 30 minutes post burn, normalises over 3 hours (anomalous); in non-burn, Pc ↓
- Pif becomes highly negative (from –1 to –100 mmHg); tissue compliance changes and denaturation of collagen
- σ range 0–1; normal 0.85–0.95; in burn 0.45
- πc normally 25–30 mmHg, ↓ to half; protein loss and dilution by crystalloid resuscitation
- πif normally 10–15 mmHg, slight ↑ in burn area
Figure S46.3 The Landis–Starling equation, where Jv is the volume of fluid moving out of the microvasculature, Kf is the capillary filtration coefficient, Pc and Pif represent capillary/interstitial fluid hydrostatic pressure, σ is the osmotic reflection coefficient, and πc and πif represent capillary/interstitial fluid oncotic pressure.
The use of Skin Grafts and Skin Substitutes
Until very recently, the early definitive closure of wounds proved problematic when full-thickness burns exceeded 50% of the TBSA. The mainstay of burn wound repair has been the split-skin autograft and, at >50% TBSA, the burn area exceeds the donor site area. A number of manoeuvres have been established to facilitate coverage of these wounds by grafting, all of which are utilised in patients with the most extensive burn wounds. Techniques include serial episodes of grafting surgery, harvesting very thin autografts (to allow more rapid re-epithelialisation of the donor sites, facilitating earlier reharvest and allowing a greater number of harvests from the same donor site), widely meshing the grafts or using a Meek–Wall technique (Meek, Humeca, Enschede, the Netherlands). This latter technique involves using small pieces of graft, placed in a specialised holder on a cork board and run through a series of blades perpendicular to each other, to create small squares of graft each 3 mm × 3 mm. Once cut, the holding platform can be pulled apart (to differing distances – the ‘mesh ratio’), separating the tiny grafts. Although ‘fiddly’ and laborious, this technique minimises graft wastage, since even small pieces of graft can be meshed in this way. The use of cadaver skin to cover the non-grafted wounds pending donor site re-epithelialisation and ‘reharvestability’ gained popularity in the late twentieth century as issues of consent and techniques for harvest and storage (banking) were refined.
The use of cadaver skin has a number of limitations. Skin banks are frequently short, or devoid, of stock. Its presence ‘passively’ temporises the wound, ‘buying time’ but not improving the wound bed, merely allowing undirected granulation. It cannot be used unless the patient is pathologically immune suppressed.
The dermal matrix strategy, pioneered by Jack Burke, sought to redress some of these issues. In producing a ‘scaffold’ to allow autologous tissue in-growth and establish a ‘neo-dermis’ (‘active’ temporisation), he improved the outcome of the thin, meshed skin graft. A completely synthetic, biodegradable polymer version has also been developed (Figures 46.9 and 46.10).
However, the material Burke (and Ioannis Yannas) produced (Integra Dermal Regeneration Template, Integra Lifesciences, Plainsboro, NJ, USA), which is a cross-linked bovine type I collagen scaffold supported by shark fin chondroitin-6-sulphate glycosaminoglycan, is physiologically closed with a bonded pseudo-epidermis of silicone and the length of the processing time to manufacture the material renders it expensive. The expense of the product and issues arising from placing non-vascularised biological material on the surface of a wound in an immunocompromised patient, and expecting neovascularisation to occur before infection, has resulted in its varying usability – some surgeons have had success and others not. Because of the cost, those surgeons who can make it work tend to limit its use to the biggest burns, and then only use it as a ‘patch-up’ to cover the wounds that remain following the primary grafting procedure. In this situation, the material is being used to temporise because it physiologically closes the wound, reducing inflammation/proliferation and contraction. Since its integration improves the wound bed for subsequent closure, we can consider it to be an ‘active’ temporiser. The long-term in vivo histology of these materials demonstrates that the exogenous collagen is absorbed and replaced by autologous collagen. The exogenous collagen thus provides a ‘scaffold’ for fibroblast ingress and neovascular growth, analogous to the scaffolding used by builders, i.e. it is removed once the ‘job’ is complete. A second analogous principle can be derived from the builders – the workmen do not move ‘in’ scaffolding pipes and decking boards, but within the spaces in between. Once this principle is grasped – that it is the spaces within the scaffold that are important – the ‘temporary’ scaffolding itself becomes less important, and thus does not have to be of biological origin but can be made of any biodegradable material as long as it is biocompatible, bio-tolerated and demonstrably safe (not cytotoxic, carcinogenic or teratogenic). Growing environmental pollution has forced the development of biodegradable polymers and, at the start of this millennium, a completely synthetic, biodegradable polyurethane dermal matrix was designed and developed by Professor John Greenwood and team, in Adelaide, Australia, using biodegradable polymer developed in Melbourne, Australia; this is known as the NovoSorb Biodegradable Temporising Matrix (BTM; Polynovo).
During the development of this material, several unexpected outcomes were observed. The synthetic composition means that it is not prone to attack by microorganisms (it is not a ‘foodstuff’). Thus, infection is uncommon; if it does occur, it is localised and manageable without loss of the matrix.
Increasing burns use also demonstrated that loss of skin graft over the integrated BTM is rare and that the appearance of the autograft mesh pattern (even with widely meshed graft) is considerably better than autograft alone. This finding has also been observed with collagen-based matrices and has its basis in the principles of wound healing.
The dermal substitute is allowed to integrate into the burn wound, a process that can take 3–6 weeks. Once integrated the bed can be considered a ‘neo-dermis’ of ordered granulation tissue and a split-skin graft is placed.
The presence of a ‘neo-dermis’ provides a bed across which interstitial epithelialisation can occur without needing granulation tissue and so the cosmetic appearance is improved. In fact, the thinner the graft, the better the appearance and the less obvious the mesh pattern.